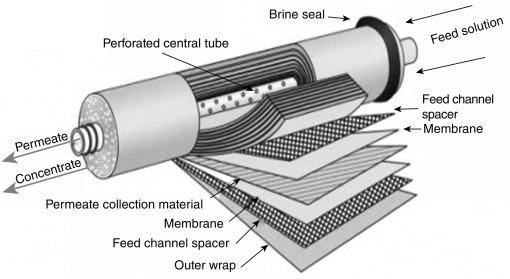
Reverse osmosis (RO) is a fundamental method for addressing a global water scarcity. Its ability to eliminate contaminants, minerals, and salts qualifies it for industrial usage, wastewater treatment, and potable water. Generally polymeric thin films, traditional RO membrane materials have permeability, fouling resistance, and energy consumption problems. Search is for creative materials to solve these issues and provide more affordable, environmentally friendly, and efficient water filtration devices. Their enhanced performance and capacity to meet world clean water demands are driving prioritizing for nanomaterials and complex polymers As these new materials are developed, RO technology could revolutionize water treatment all around.
The limits of conventional thin film composite membranes
Thin-film composite (TFC) architecture with a polyamide (PA) active layer, polysulfone (PSf) support layer, and non-woven fabric backing makes up traditional RO membranes. Cheaper and suitable for mass production are polymeric materials. They do, nonetheless, have some drawbacks. Fouling—the accumulation of organic and inorganic pollutants on the membrane surface—which requires constant and costly cleaning—reduces their permeability. Still another big problem is the permeability-selectivity trade-off. Reducing membrane thickness will increase permeability, hence decreasing desired solute rejection. These membranes also have low mechanical strength, which lowers their lifespan and calls for regular replacement. To solve these limitations, therefore, membrane materials with improved permeability, selectivity, fouling resistance, and stability are under development. Ultimately, by lowering energy and waste, RO membranes should be more environmentally friendly and efficient.
Creative Two-Dimensional Material: graphene
Potential reverse osmosis membrane materials include graphene, a hexagonal lattice of carbon atoms. For next-generation membranes, its superb mechanical strength, surface area, electrical and thermal conductivity, and mechanical integrity fit. Thanks to its atomic thickness, hydrophobic graphene lowers fouling and generates high-permeability membranes. Moreover, its robust architecture increases membrane lifetime. Scientists are looking at methods to include graphene oxide (GO) nanosheets to the active or support layer of a TFC membrane into RO membrane designs. While selectively rejecting salts, nanochannels between GO sheets improve water permeability. Maintaining high rejection rates and the scalability and cost-effectiveness of graphene manufacture depend on control of nanochannel generation. Research is rapidly resolving these challenges; graphene has the potential to revolutionize RO membrane technology even with them.
Strength of Carbon Nanotube Utilization
Another kind of carbon nanotubes (CNTs) offer unique characteristics that help RO membrane development. Great mechanical strength, aspect ratio, and customisable surface properties are obtained by rolling graphene sheets into cylindrical CNTs. Their highly defined pores provide them somewhat permeable, selective membranes. CNT membranes might provide a basis for various filtering materials, embedded in polymer matrices, or vertically aligned. Scholars are investigating these approaches. Vertically oriented CNT membranes provide significant water permeability due low nanotube flow resistance. CNTs increase anti-fouling and mechanical strength of polymer composite. Like graphene, uniform CNT dispersion in the polymer matrix and nanotube diameter and orientation still challenge us. Though manufacturing and alignment problems exist, carbon nanotubes show great potential for high-performance RO membranes.
Modern Polymer Development: Membrane Optimization
Polymer chemistry is enabling fresh RO membrane performance paths outside of nanoparticles. Researchers are building polymers with specific properties to get around conventional material constraints. This entails creating mechanical strength, chemical stability, anti-fouling polymers. Minimizing membrane fouling, synthesizing polymers with zwitterionic groups repels proteins and other organic molecules. Improved selectivity may come from new hyper-branched polymers with superior pore size and dispersion control.